The electromagnetic spectrum is the full range of all frequencies of electromagnetic radiation, ranging from low-frequency, long-wavelength radio waves to gamma rays, characterized by extremely high frequencies and short wavelengths. This spectrum is classified into different ranges or bands according to their frequencies, and each has unique applications in our daily lives and in scientific development. For example, radio waves are used in communications and broadcasting, while microwaves have applications in telecommunications and home technology. As we move up in frequency, we find visible light, ultraviolet rays, X-rays and finally gamma rays, each with specific properties and effects that make them useful in fields such as medicine, astronomy and nuclear physics.
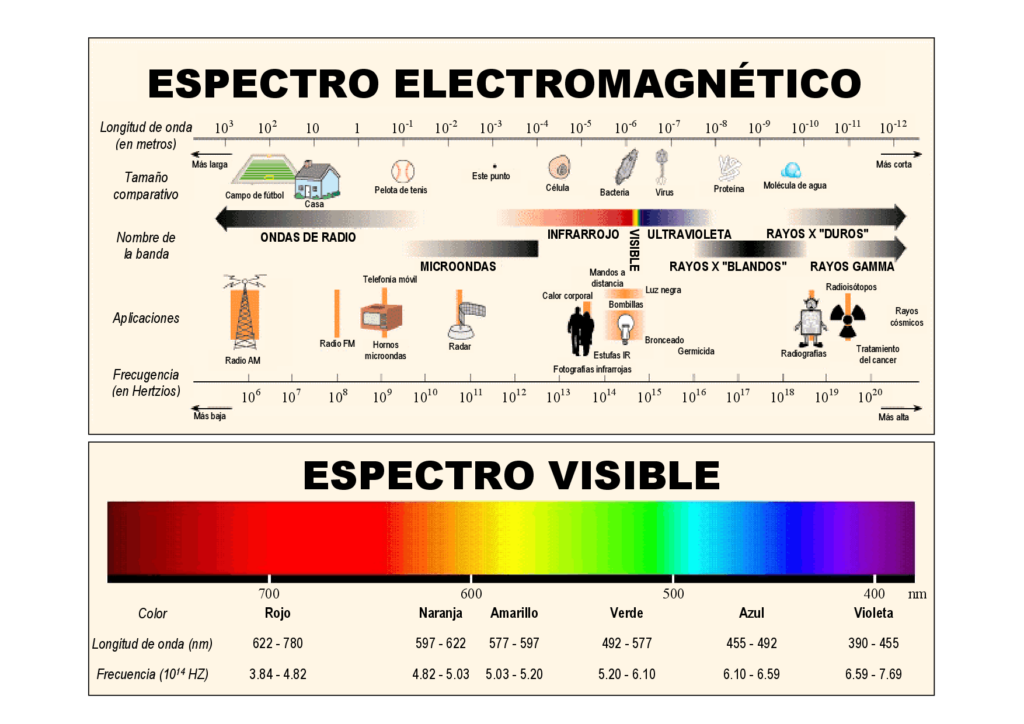
Having clear conceptually what is the electromagnetic spectrum, it is necessary to keep in mind the following concepts:
Amplitude, Frequency and Phase.
Amplitude:It is the height of the wave and represents the signal strength. In AM, the amplitude of the carrier wave varies according to the information signal.
Frequency: The number of wave cycles per second, measured in hertz (Hz). In FM, the frequency of the carrier wave changes to encode the data.
Phase: Refers to the position of a point in the wave cycle. In PM, the phase of the carrier wave is altered to transmit the information.
Let’s look at an example.
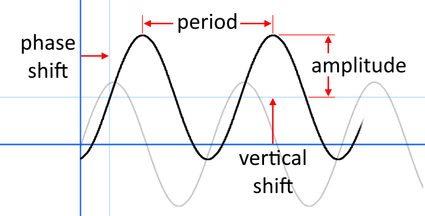
Radio waves
Radio waves are a type of electromagnetic radiation with relatively long wavelengths and low frequencies. They are a form of energy that propagates through space in the form of oscillating electric and magnetic fields. Radio waves are commonly used for wireless communication, broadcasting and various scientific and technological applications.
They are generated when charged particles, such as electrons, oscillate or accelerate in antennas and other devices. These oscillations create changing electric and magnetic fields that propagate through space.
Radio waves have several important characteristics that make them suitable for a variety of applications:
Long-distance propagation: radio waves can travel long distances, even above the curvature of the earth. This property makes them ideal for long-distance communication and transmission.
Penetration and diffraction: Radio waves can pass through obstacles such as walls and buildings, allowing them to be used for indoor communication. They also diffract around obstacles, which helps them reach receivers that are not in line of sight.
Wide frequency range: Radio waves cover a wide frequency spectrum, ranging from a few kilohertz (kHz) to several gigahertz (GHz). This wide range allows for different applications, such as AM/FM radio, television broadcasting, satellite communication and wireless networks.
Low energy and non-ionizing: Radio waves have relatively low energy compared to other forms of electromagnetic radiation, such as X-rays or gamma rays. They are considered non-ionizing, meaning that they do not have enough energy to extract electrons from atoms or molecules, making them generally safe for human exposure.
Penetration and diffraction AB
Penetration and diffraction are two important characteristics of radio waves that contribute to their ability to propagate through obstacles and reach receivers even when there is no direct line of sight between the transmitter and receiver.
Penetration: Radio waves have the ability to penetrate certain obstacles, such as walls, buildings and vegetation. The degree of penetration depends on the frequency of the radio waves and the nature of the obstacle. Lower frequency radio waves tend to have better penetration capabilities compared to higher frequency waves.
When radio waves encounter an obstacle, such as a wall, some of the energy of the wave can pass through the obstacle due to diffraction and the ability of the electromagnetic wave to couple with the conductive materials of the obstacle. The penetrating ability of radio waves makes them suitable for indoor wireless communication, such as Wi-Fi networks, where signals can propagate through walls and other obstructions.
Diffraction: is the deflection and propagation of radio waves when they encounter an obstacle or pass through an opening of comparable size to their wavelength. When radio waves encounter an edge or obstruction, such as a building or mountain, they diffract around it, allowing the wave to reach areas beyond the direct line of sight.
Diffraction occurs because radio waves exhibit wave-like behavior, similar to other waveforms. When the waves encounter an obstacle, they scatter, creating a pattern of secondary waves propagating in different directions. This phenomenon allows radio waves to “bend” around corners and reach receivers that are not in the direct path of the transmitter.
The amount of diffraction depends on the wavelength of the radio waves and the size of the obstacle relative to the wavelength. Radio waves with longer wavelengths, such as those used in AM broadcasting, can diffract more easily around larger obstacles. In contrast, radio waves with shorter wavelengths, such as those used in Wi-Fi networks, experience more limited diffraction effects.
Diffraction is especially important for radio waves in urban environments, where buildings and other structures can obstruct the direct line of sight between the transmitter and receiver. By taking advantage of the diffraction phenomenon, radio signals can reach receivers located behind buildings or around corners, allowing communication over a wider area.
Both penetration and diffraction play a key role in ensuring the reliability and coverage of radio communication systems. By allowing radio waves to propagate through and diffract around obstacles, they enable wireless communication in a variety of scenarios and environments.
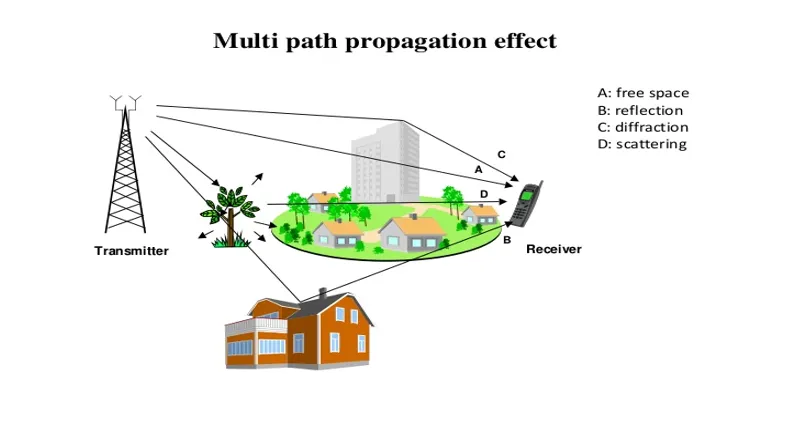
AD Low energy and non-ionizing
Radio waves are characterized by their low energy compared to other forms of electromagnetic radiation, such as X-rays or gamma rays. This low energy level is an important characteristic of radio waves and contributes to their safety and non-ionizing nature.
Low energy: The energy of a radio wave is directly related to its frequency. Radio waves have relatively low frequencies compared to other types of electromagnetic radiation. The energy of a photon, which is a discrete packet of electromagnetic energy, is given by Planck’s equation: E = hf, where E represents the energy, h is Planck’s constant and f is the frequency of the wave. Since radio waves have low frequencies, the energy of their photons is correspondingly low.
Non-ionizing: The term “ionizing radiation” refers to forms of electromagnetic radiation that have sufficient energy to extract electrons from atoms or molecules, thus ionizing them. Examples of ionizing radiation are X-rays, gamma rays and some ultraviolet (UV) radiation. In contrast, radio waves do not have sufficient energy to cause ionization. When radio waves interact with matter, they generally do not have the ability to break molecular bonds or produce ions.
The non-ionizing nature of radio waves is an important factor in terms of their impact on biological tissues and their safety for human exposure. Unlike ionizing radiation, which can cause damage to cells and DNA, radio waves are considered biologically safe at typical exposure levels. This makes radio waves suitable for a wide range of applications, including wireless communications, broadcasting and various technologies without posing significant health risks.
It is important to note that while radio waves are non-ionizing and generally considered safe, very high power or prolonged exposure to radio waves can cause localized heating effects. This is the principle behind techniques such as diathermy, which uses high-frequency radio waves for therapeutic heating in medicine. However, for typical levels of everyday exposure to radio waves from common sources such as wifi, cell phones and radio transmissions, the energy is well below the threshold that causes significant heating or biological damage.
Regulatory agencies around the world, such as the Federal Communications Commission (FCC) in the United States, establish radio wave exposure guidelines and limits to ensure public safety. These guidelines take into account known biological effects and are designed to provide a margin of safety.
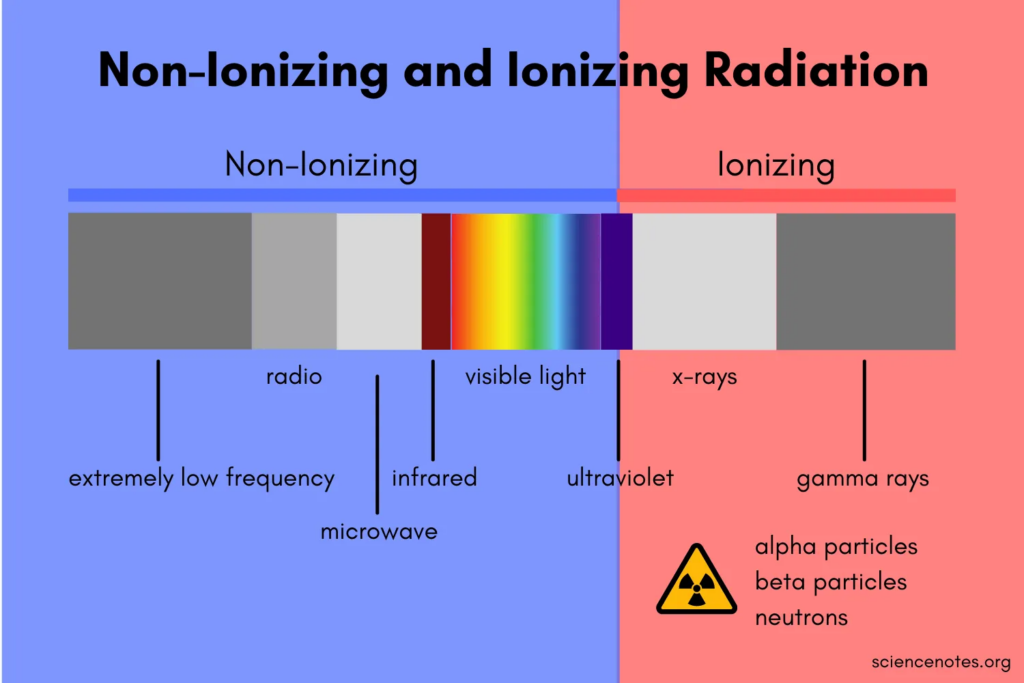
Length of radio waves
The length of radio waves is measured in meters (m) or multiples of these, such as kilometers (km) or millimeters (mm), depending on their frequency. Since radio waves have a wide range of frequencies, their lengths can vary significantly.
Radio waves cover a wide spectrum of frequencies, typically ranging from a few kilohertz (kHz) to several gigahertz (GHz). This frequency range corresponds to wavelengths ranging from hundreds of kilometers to a few millimeters. The longer the wavelength, the lower the frequency and vice versa, according to the inverse relationship described by the equation:
c = λ * f
Where:
- c – represents the speed of light in vacuum (approximately 3 x 10^8 meters per second).
- λ – represents the wavelength of the radio wave
- f – represents the frequency of the radio wave
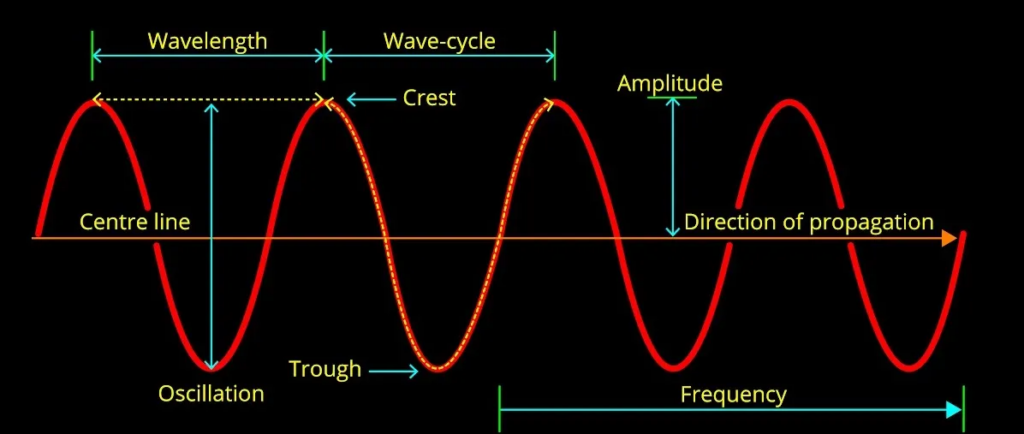
For example, at a frequency of 1 megahertz (MHz), the wavelength is about 300 meters. At higher frequencies, such as 1 gigahertz (GHz), the wavelength is much shorter, about 30 centimeters.
Knowing the wavelength of radio waves is important for several reasons:
- Antenna design and performance: antenna design and performance depend on the wavelength of the radio waves they are intended to transmit or receive. Antennas are typically designed to be resonant at specific wavelengths for efficient transmission or reception. By understanding the wavelength, engineers can design antennas that are correctly matched to the desired frequency range, optimizing their performance and ensuring effective communication.
- Propagation characteristics: The wavelength of radio waves directly affects their propagation characteristics. Longer wavelengths tend to diffract more around obstacles, allowing them to propagate over longer distances and penetrate buildings more effectively. On the other hand, shorter wavelengths exhibit more directional propagation and are more susceptible to absorption and reflection by objects in their path. Understanding wavelength helps predict how radio waves will behave in different environments, which is crucial for planning wireless communication systems and optimizing signal coverage.
- Frequency selection and interference prevention: The wavelength is inversely proportional to the frequency of a radio wave. Knowing the wavelength makes it easier to determine the corresponding frequency or vice versa. This is important for frequency selection and coordination in various applications to avoid interference with other wireless systems operating in the same or nearby frequency bands.
- Regulatory compliance: Regulatory agencies, such as the FCC, assign specific frequency bands for different applications and set limits on maximum allowable power levels. These regulations often refer to wavelength or frequency range. Knowing the wavelength helps users and equipment manufacturers to ensure compliance with regulatory requirements and to respect the frequency bands assigned for specific applications.
- Compatibilidad e interoperabilidad: los distintos dispositivos y sistemas inalámbricos funcionan dentro de rangos de frecuencia o bandas de longitud de onda específicos. Al comprender la longitud de onda, resulta más fácil evaluar la compatibilidad e interoperabilidad de varios dispositivos y sistemas. Por ejemplo, al integrar diferentes tecnologías inalámbricas o planificar implementaciones de redes, el conocimiento de la longitud de onda ayuda a garantizar que los dispositivos y sistemas funcionen dentro de rangos de frecuencia compatibles para evitar interferencias y lograr una comunicación fluida.
To better understand how it works, let’s calculate the wavelength for FM radio – 100 MHz, Smart Home – 433.92 MHz, Wi-Fi and GSM:
Radio FM 100 MHz:
λ = (3 x 10^8 m/s) / (100 x 10^6 Hz)
λ = 300'000'000 / 100'000'000
λ = 3 meters
Smart home 92 MHz:
λ = (3 x 10^8 m/s) / (433,92 x 10^6 Hz)
λ = 300'000'000 / 433'920'000
λ ≈ 0,691 meters or 69,1 centimeters
Wi-Fi operates in the 2.4 GHz and 5 GHz frequency bands. Let’s calculate the wavelengths for both:
For 2.4 GHz:
λ = 3 x 10^8 m/s / 2,4 x 10^9 Hz
λ = 300'000'000 / 2'400'000'000
λ ≈ 0,125 meters or 12,5 centimeters
For 5 GHz:
λ = 3 x 10^8 m/s / 5 x 10^9 Hz
λ = 300'000'000 / 5'000'000'000
λ ≈ 0,06 meters or 6 centimeters
GSM: The global system for mobile communications operates in several frequency bands. Let us calculate the wavelength for the most common GSM frequency band, which is 900 MHz:
λ = (3 x 10^8 m/s) / (900 x 10^6) Hz
λ = 300'000'000 / 900'000'000
λ ≈ 0,333 meters o 33,3 centimeters
Modulation
Modulation is the process of altering a carrier wave to transmit information. It can change the amplitude, frequency or phase of the carrier wave.
We can see a summary of some types of modulations.
- Amplitude modulation(AM): is a modulation technique in which the intensity or amplitude of a carrier signal is varied to transmit information. It is commonly used in broadcasting.
- Frequency Modulation(FM): In FM, the frequency of the carrier signal changes according to the information being transmitted. It is known for its high quality audio and is used in FM radio broadcasting.
- Phase Modulation(PM): PM involves changing the phase of the carrier signal in response to the information being transmitted. It is used in a variety of applications, including digital communication systems.
- Quadrature Amplitude Modulation( QAM ): QAM is a type of modulation that combines both amplitude and phase modulation. It allows the transmission of multiple bits of information simultaneously, making it efficient for high-speed data transmission, such as in Wi-Fi and cellular communications.
- Quadrature phase shift keying( QPSK): QPSK is a digital modulation scheme in which two bits of information are encoded in the phase of the carrier signal. It is widely used in satellite communications and digital broadcasting.
- Binary phase shift keying( BPSK): BPSK is a type of modulation in which the phase of the carrier signal is shifted to represent binary data. It is commonly used in wireless communication systems.
- Orthogonal Frequency Division Multiplexing( OFDM): OFDM is a multicarrier modulation technique that divides the available frequency spectrum into multiple subcarriers. It is used in many modern communication systems, including Wi-Fi and 4G/5G cellular networks.
- Amplitude Shift Keying (ASK): is a modulation technique in which the amplitude of the carrier signal is changed to represent digital data. It is simple and used in applications like RFID and optical communication.
- On-Off Keying (OOK): is a specific form of ASK where the carrier signal is turned on and off to represent binary data, with “on” representing a 1 and “off” representing a 0. It is commonly used in remote controls and low-power communication systems.
Finally, the fundamental concepts of the spectrum meter and radio frequencies in general terms have been introduced.
Leave a Comment